Case Study
Source or Sink? Inland Waters Can Be Both for Nitrous Oxide
May 2, 2024
Freshwater lakes and streams are natural sources of nitrous oxide (N2O) in the atmosphere, a potent greenhouse gas. A recent study using data from NEON aquatic sites shows that across the continent, under the right conditions, these lakes and streams may also act as N2O sinks. This sink behavior may help to partially offset N2O emissions from freshwater sources and is crucial to account for in climate modeling.
Dr. Kelly Aho, an Assistant Professor in the Department of Earth and Environmental Science and Integrative Biology at Michigan State University and a former NEON Postdoctoral Fellow, led the study, which was recently published in Geophysical Research Letters: “Inland Waters Can Act as Nitrous Oxide Sinks.”
Why Care About Nitrous Oxide?
Nitrous oxide is a colorless, non-flammable gas produced by both natural and anthropogenic (human-generated) processes. N2O is sometimes used as an anesthetic and analgesic in medical and dental procedures, with euphoric effects that have earned it the nickname “laughing gas.” Its impact on the environment, however, is no laughing matter. N2O is a greenhouse gas that is significantly more potent, pound for pound, than carbon dioxide and has a long atmospheric lifetime. It also contributes to the depletion of the ozone layer.
The flux of N2O between the atmosphere and terrestrial and aquatic systems is closely tied to the nitrogen cycle. Nitrogen gas (N2) makes up roughly 78% of the atmosphere, and is an essential nutrient for all living organisms. Nitrogen is continually cycling between different chemical forms through various processes including nitrification and denitrification. The end product of nitrification is nitrate (NO3)—a primary nutrient source for plant growth. In denitrification, NO3 is reduced to N2 gas by anaerobic bacteria in soil and water and the decomposition of organic matter. Denitrification removes excess nitrate from soil and water, preventing it from leaching into groundwater and reducing the risk of eutrophication (nutrient overload) in aquatic environments. However, this reaction does not always reach completion; when this happens, N2O is released along with N2.
While N2O is released by many natural processes, human activities—including the use of synthetic fertilizers for agriculture, fossil fuel combustion, large-scale livestock farming, and deforestation—have affected the natural nitrogen cycle. This has resulted in increased concentrations of N2O in the atmosphere, which contributes to global warming. Understanding the net flux of N2O between the atmosphere and terrestrial and aquatic systems is important for creating accurate models of climate change.
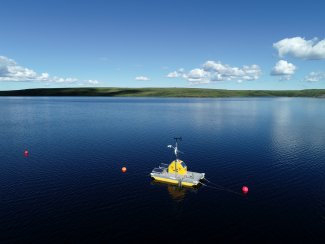
Toolik Lake Buoy, Domain 18, Alaska.
Aho explains, “I study gas exchange in inland waters because they are these hotspots in the landscape where a lot of different processes converge. They have lots of biogeochemical activity and a lot of variability in their hydrology. I’m interested in understanding this variability and the processes that control the production and flux of these gases.”
Looking at Dissolved Gas Concentrations at NEON Aquatic Sites
Nitrogen enters aquatic systems through runoff and soil leaching, wastewater discharge, precipitation, and other processes on land. “Streams, rivers, and lakes act as drainage networks,” says Aho. “They’re kind of like gutters on the landscape; they get a lot of flushing of nutrients and chemicals from terrestrial systems.”
Freshwater lakes and streams are generally considered sources of N2O to the atmosphere rather than sinks. Gases move between the atmosphere and aquatic systems across the water boundary, which acts like a membrane. If the concentration of N2O in the water is higher than the atmospheric concentration, N2O gas molecules will diffuse into the atmosphere across the water boundary until an equilibrium is reached.
But under certain circumstances, the water can act as a sink. If the water is undersaturated with N2O—that is, if the atmospheric concentrations are higher than the water concentrations—the body of water will instead pull N2O gas out of the atmosphere.
Climate models that assume lakes, rivers, and streams are always just sources of N2O may be overestimating the amount of N2O they add to the atmosphere on an annual basis. Localized field studies have documented undersaturation of N2O in various aquatic ecosystems; Aho detected it in the Connecticut River Watershed while working on her Ph.D. However, there were no studies examining the phenomenon at larger scales.
The NEON dataset, which collects dissolved gas data at sites across the continent, provided an opportunity to look at gas exchange in many different ecosystems across longer time periods. “NEON data let me think about how widespread this phenomenon might be,” says Aho. “That’s what is so powerful here—the potential to upscale findings to the regional and continental scale.”
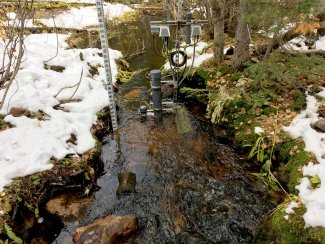
Stream sensors at COMO in the snow
Aho and her co-authors used data from NEON’s 34 aquatic field sites which include seven lakes, 24 wadable streams, and three non-wadable rivers spanning many latitudes and biomes from the tundra in northern Alaska to the neotropics in Puerto Rico. Using the dissolved gases in surface water data product, along with paired air samples, they were able to determine whether the waters were over- or undersaturated with N2O compared to the atmosphere (and thus whether they were acting as sources or sinks at the time). NEON collects measurements monthly from the lake sites and two times per month from rivers and streams.
The study confirmed that most of the time, inland waters at NEON field sites are slightly oversaturated with N2O, making them net sources of N2O to the atmosphere. However, undersaturated states—times when lakes, streams, and rivers would be acting as nitrous oxide sinks—were observed at nearly all NEON sites. These observations crossed all latitudes and biomes, though the amount of time spent in an undersaturated state varied across field sites.
Estimating N2O Emissions from Inland Waters
The study suggests that current climate models may be overestimating the total annual volume of N2O that freshwaters add to the atmosphere by ignoring the times when they may be acting as sinks instead of sources. Aho says, “As we think about our models of nitrous oxide emissions, we need to take into account that undersaturation does, in fact, occur across all of the latitudes and biomes we studied. To avoid overestimating the flux rate, we need to consider when and how often this is happening and how much nitrous oxide inland waters sequester during these times. Thinking about emissions from inland waters globally, people will need to take this sink behavior into account and how it might offset total emissions.”
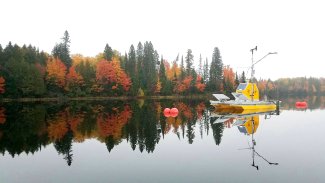
A buoy on the CRAM lake site in the fall
However, more work remains to be done to estimate the scale of this effect. This study did not point to any clear patterns in undersaturation vs. oversaturation based on location, season, temperature, precipitation, or other easily identified variables. One hypothesis is that undersaturation may be related to low overall rates of nitrification and denitrification at a given point in time. Aho also points out that the NEON aquatic sites are not representative of all inland aquatic sites globally, as they tend to be located in areas with less human impact. Understanding the drivers of source and sink behavior in inland waters will require more study, including analysis of a wider range of aquatic systems using more frequent sampling.
Aho is continuing to work with the NEON dissolved gas data, building off work that began during her Ph.D. research and her postdoctoral studies with NEON. For her next analysis, she plans to look at dissolved carbon dioxide, methane, and nitrous oxide together for any linkages or correlations between them. She is also studying an aquatic system in Michigan with more frequent sampling in hopes of developing a more process-based understanding of N2O emissions. “One of the takeaways of this work is that it’s really important to think about temporal variability,” she says. “You can’t just take a snapshot in time. You have to think about how these sites vary through time with seasons, different rainfall patterns, and other variables. Understanding those variable timescales will give us insight into the processes and drivers of this behavior. That, in turn, will help us upscale these estimates for a better picture of emission rates from aquatic systems globally.”